Background
Electronic skins, e-skins for short, is innovative soft electronics technology that gives robots and devices tactile sensing capabilities like human touch and feel. Robots equipped with human touch sensitivity could be deployed in many different applications ranging from manufacturing and food handling/preparation to medical care and surgery, where the ability to assist in surgical procedures or providing nursing care such as checking a patient’s pulse or performing a CPR (cardiopulmonary resuscitation) is beneficial and even life-saving. Assisted or in-home care is ideally suited for robot nurses or physical therapists equipped with the kind of softness and touch sensitivity as a human hand.
Existing e-skin technology loses sensing accuracy as the material stretches. Providing a stretchable e-skin that can mimic the softness and deformability of human hands, while maintaining accurate pressure-sensing capabilities, has been intensively researched over a couple of decades. Capacitive pressure sensors have been spotlighted due to their high sensitivity, superior repeatability and stability, and simple construction. Soft capacitive pressure sensors using stretchable electrodes and dielectric materials have been developed.
However, innovations in materials and structures continue to have an intrinsic bottleneck of losing sensitivity to both in-plane stretch and out-of-plane pressure. When the material is subjected to stretch and pressure is applied simultaneously, it is difficult to discriminate the source of capacitance change and hence to provide accurate pressure reading under stretch. Existing techniques, including stiff-island-enable strain isolation or signal compensation through additional strain sensing, have drawbacks and add complexity.
This invention from researchers at The University of Texas at Austin shows an intrinsically stretchable capacitive pressure sensor that is impervious to stretch yet highly sensitive to pressure.
Technical description
The University of Texas at Austin researchers have invented Stretchable Hybrid Response Pressure Sensors (SHRPS) consisting of four stretchable layers, including two carbon nanotube (CNT)-embedded polydimethylsiloxane (PDMS) as electrodes, barely electrically conductive porous nanocomposite (PNC), and a PDMS insulating layer. See Figure 1A. The PNC is composed of Ecoflex and CNT, and the CNT doping ratio is slightly higher than the percolation threshold which makes the PNC barely conductive. The PNC is an open-cell structure with 82% porosity, allowing for dispersed parasitic capacitance in the PNC. The entire device becomes capacitive by placing a 2 μm-thick insulating PDMS layer between the PNC and one side of the electrode.
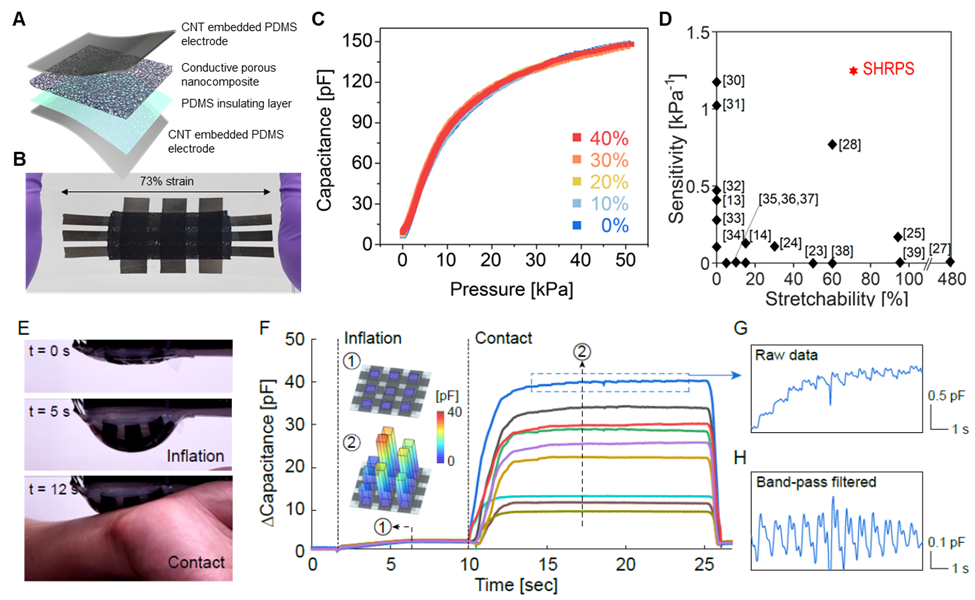
Figure 1. Stretchable hybrid response pressure sensors (SHRPS). (A) Schematic representation of the simple four-layer lamination to form a SHRPS.
(B) A 3 x 3 array of SHRPS under stretching.
(C) The pressure response of SHRPS is impervious to different stretches indicated by different colors of the curves.
(D) SHRPS stands out in terms of both sensitivity and stretchability compared to existing capacitive pressure sensors.
(E) SHRPS mounted on an inflatable probe that can gently touch human wrist.
(F) SHRPS have minimal responses to inflation but much more significant responses upon the contact with human wrist. Radial pulse waveforms are visible after filtering SHRPS readings.
The PNC and PDMS laminate can be sandwiched between two orthogonal groups of electrodes to form a 3x3 sensor array that can be highly stretchable. See Figure 1B. When pressure is applied, the resistance of the PNC decreases as ligaments of PNC contact each other, and the capacitance increases due to the reduced air pore sizes. The pressure-sensitive resistance and capacitance of PNC determine the total impedance of the sensor, and such hybrid responses generate a large change in the impedance of the sensor under pressure. However, when the sensor is subjected to stretch, the resistance and capacitance only undergo minor changes, resulting in almost stretch impervious e-skin. See Figures 1C. Figure 1D highlights the combined high sensitivity and high stretchability of SHRPS. The 3x3 SHRPS array can be mounted on an inflatable probe to mimic the shape and softness of a human finger. See Figure 1E. During inflation, the change of SHRPS capacitance is very small but upon touching human skin, the SHRPS capacitance rises significantly, while still maintaining high enough sensitivity to discern the subtle surface pulse waves, like a traditional Chinese medicine doctor. See Figure 1F.
Features
- Highly pressure-sensitive but stretch-impervious Stretchable Hybrid Response Pressure Sensors (SHRPS) benefiting from the complex electromechanical responses of barely conductive porous nanocomposite (PNC). See Figure 2.
- Significantly improved pressure response keeping the capacitance change for a high (40%) tensile strain to a minimal (2%) change at high pressure (50 kPa). See Figure 3.
- Excellent durability of SHRPS during cyclic pressure, tension, pressure under tensile strain and bending. See Figure 4.
Benefits
- Uncovered the mechanism and optimum carbon nanotube doping ratio for the high sensitivity of SHRPS, i.e., when it makes a transition of mechanism from the dielectric model to the SHRPS model. See Figure 5.
- Smart inflatable probe that can tune its contact geometry and stiffness to measure contact pressure such as measurement of human radial arterial pulses. See Figure 6.
- Support of inflatable gripper applications requiring firm grasping and gentle touching. See Figure 6 (I and J).
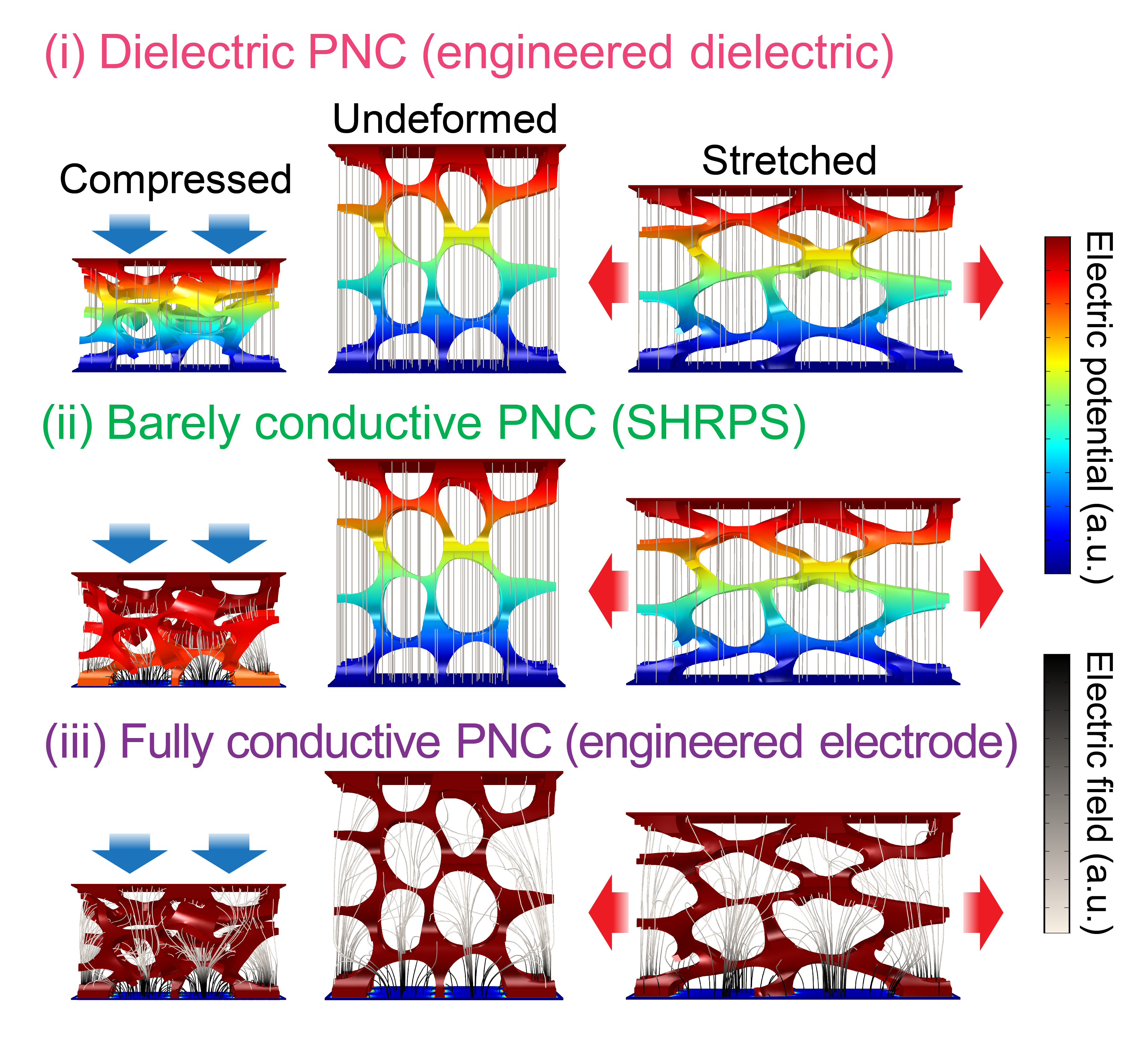
Figure 2. Finite element analysis demonstrates the unique characteristics of SHRPS (green) – pressure-sensitive but not stretch-sensitive, which differs from traditional capacitive pressure sensors that either have engineered dielectrics (pink) or enhanced electrodes (purple).
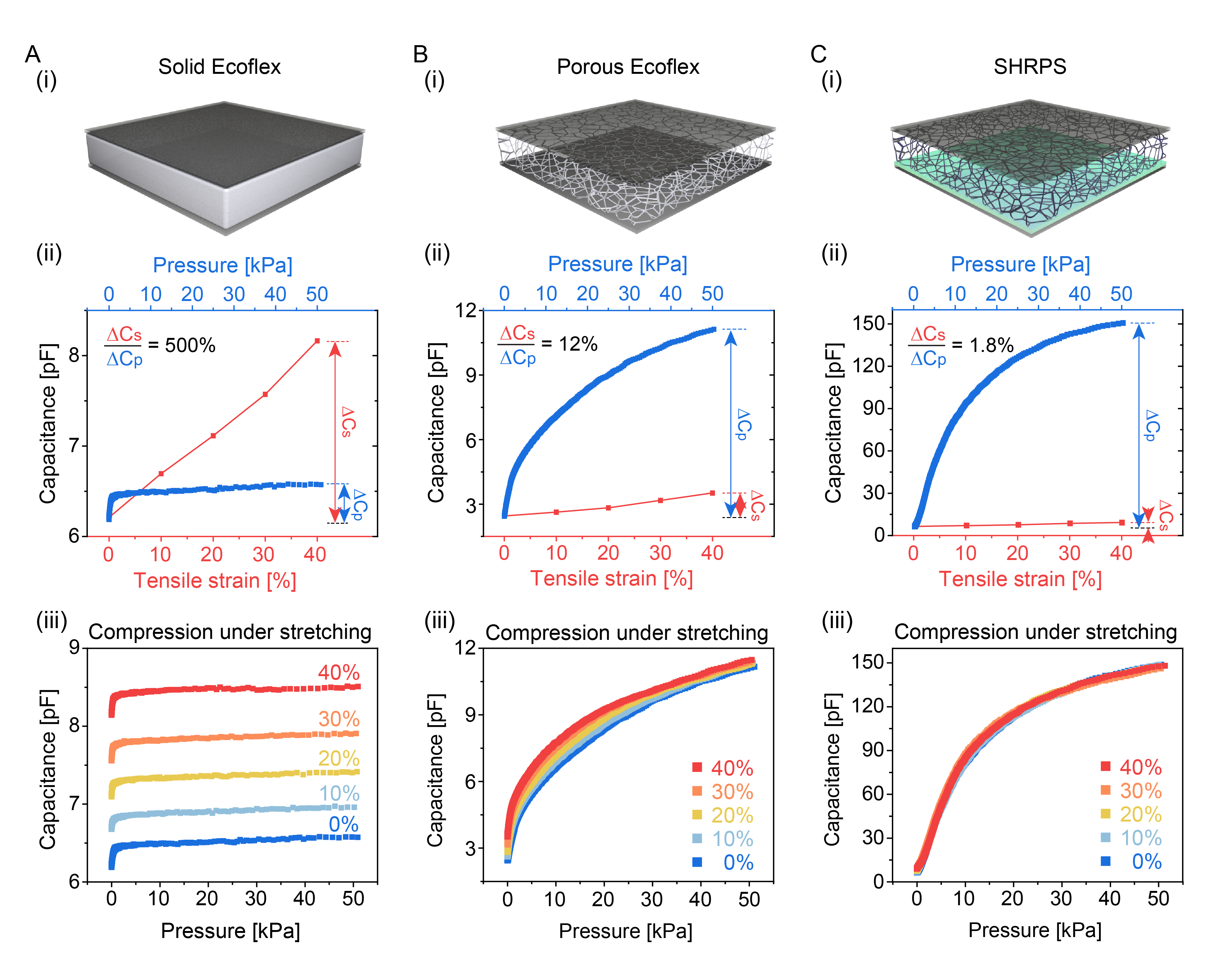
Figure 3. Pressure and stretch responses of capacitive pressure sensors with three different types of pressure sensing materials. Conventional capacitive pressure sensors with (A) solid dielectric polymer or (B) porous dielectric polymer. (C) SHRPS with barely conductive PNC and insulating layer. (A-C) (i) Schematics. (ii) Pressure (blue) and stretch (red) were applied separately. (iii) Pressure and stretch were applied simultaneously. Only the pressure responses of SHRPS are undisturbed by stretches.
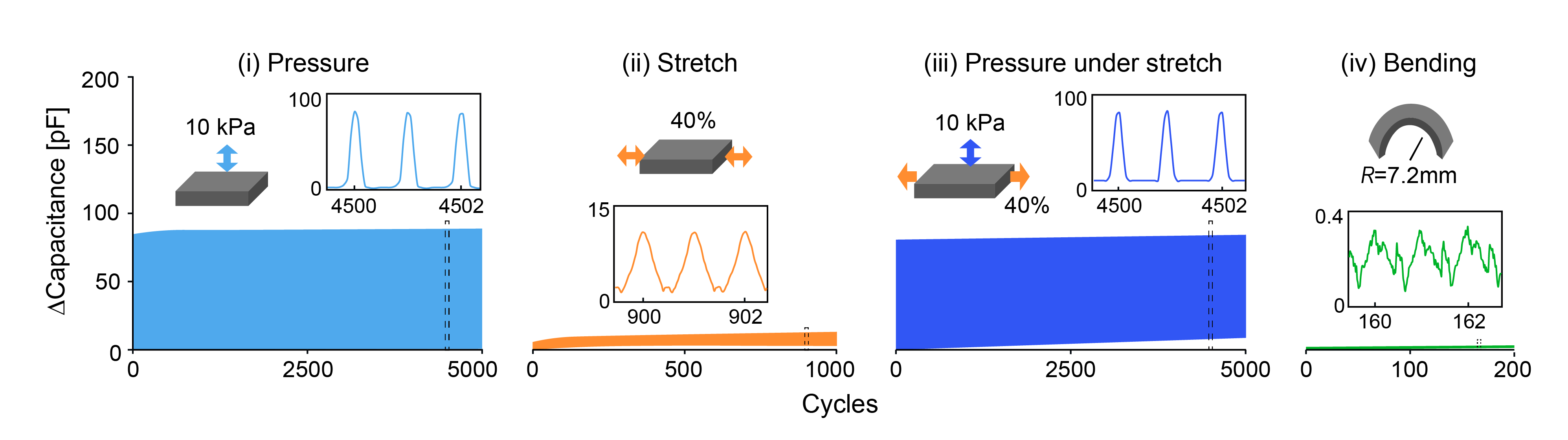
Figure 4. Repeatability and durability tests of SHRPS under i) 0-10 kPa pressure, ii) 0-40% uniaxial tensile strain, iii) 0-10 kPa pressure under a constant uniaxial tensile strain of 40%, and iv) bending from flat state to 7.2 mm radius.
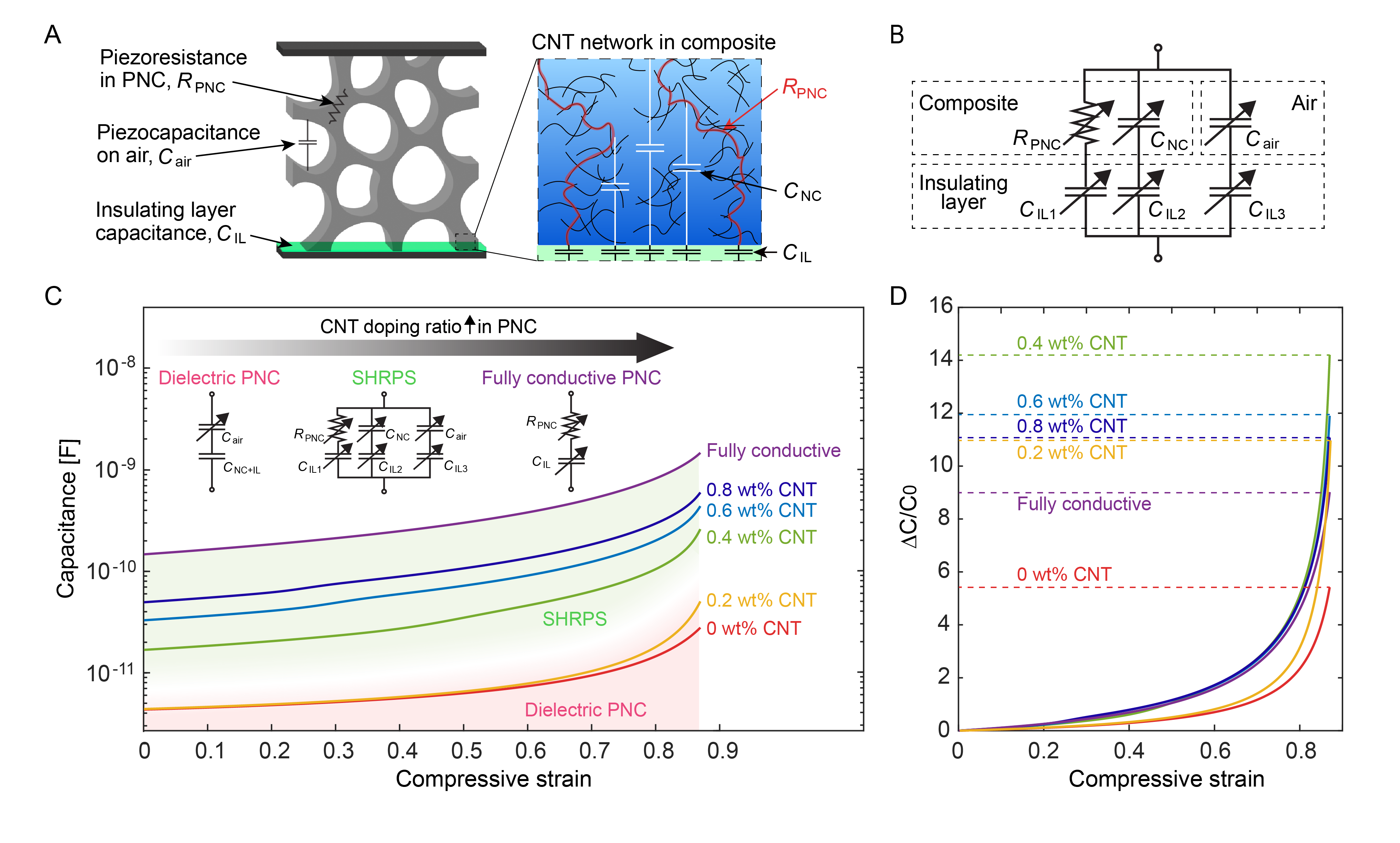
Figure 5. (A) Multiscale schematic representation of the electrical components of SHRPS. (B) Equivalent circuit of SHRPS. (C) Theoretical capacitance changes of SHRPS with different CNT doping ratios. Three different equivalent circuits were used depending on the conductivity of the PNC. (D) Normalized capacitance changes of SHRPS predicted by the model.
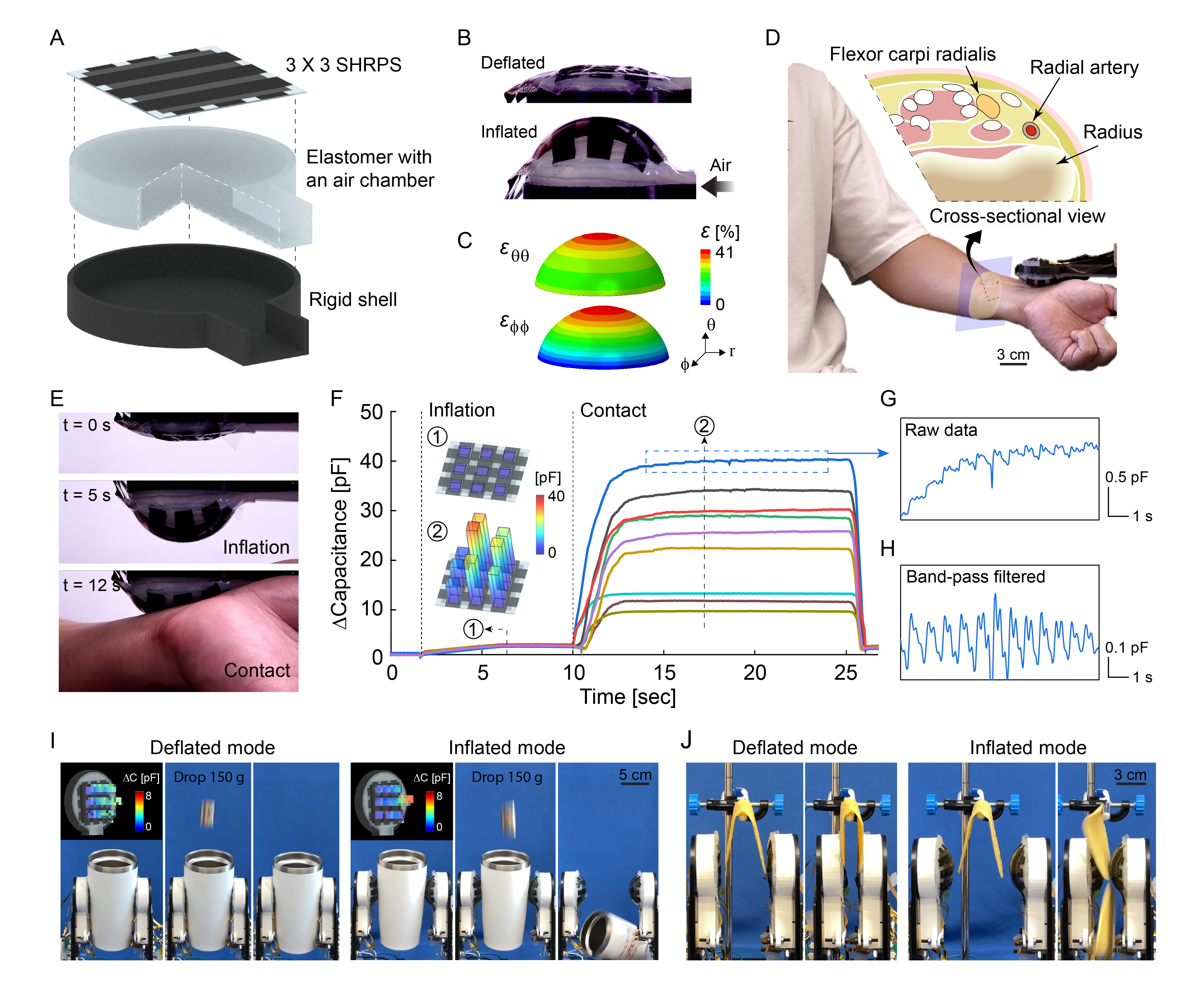
Figure 6. A 3×3 array of SHRPS on a soft inflatable probe. (A) Schematic illustration of the components of the inflatable probe. (B) The probe in deflated and inflated modes. (C) Finite element modeling (FEM) of the tensile strains in the SHRPS under inflation. (D) Photograph of the measurement of human arterial pulses using the inflated probe, with a cross-sectional illustration of the human wrist. (E) Snapshots showing the procedure of human radial pulse sensing. (F) Capacitance changes of 9 SHRPS pixels during the radial artery palpation. The two insets display the pressure distribution before (at 6 seconds) and after (at 17 seconds) contacting the wrist. (G and H) The pulse wave sensed by the central pixel. (G) The raw data and (H) the filtered data.